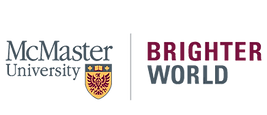
DISEASE DETECTIVES
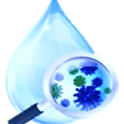
ROAD SALTS
Many of us have experienced the dread of clearing snow and ice when the cold Canadian winters arrive. The snow and ice make it hard to drive from one point to another and increase the probability of car accidents. Luckily, road salts are one major way for us to fix this problem. But, what is road salt? How does it work? Are its effects on the environment more harmful than beneficial?
The most commonly used road salt - sodium chloride - is chemically similar to regular table salt but are larger granules comparatively. Other salts such as calcium chloride and magnesium chloride are also used to increase the effectiveness of de-icing. These salts serve as excellent de-icers as they lower the freezing temperature of water and hence, prevent the water from freezing and turning into ice (Hinsdale, 2018).
Take a moment to think about how much road salt you use on a yearly basis. In Canada, around 7 million tonnes of road salt are used annually. In the United States, up to 20 million tonnes are used (Arnott et al., 2020).
There are advantages to using road salts. Road salts are cheap, convenient and very effective. They can be spread onto roadways easily either as crystalline solids or brine solutions. Road salts reduce accident rates on average 87% and 78% on two‐lane and multi‐lane highways, respectively (Hintz & Relyea, 2019).
But, there are also many disadvantages to road salts.
Chloride is extremely soluble in water and cannot be destroyed. Once applied to roads, through either surface runoff or groundwater, chloride ends up in our wetlands and other water bodies. Salt pollution changes soil composition and damages vegetation, material goods, and infrastructure, including roads and bridges. It also clogs stormwater catch basins resulting in higher potential for flooding. Salt removal from drinking water supplies requires installation of expensive desalination systems. Negative impacts of salt pollution on human health primarily include the effects from rising sodium concentrations in fresh-water drinking reservoirs, where elevated sodium levels contribute to hypertension and consequently an increased burden on health care systems. Salt pollution also affects biodiversity and the composition of microbial communities which can indirectly impact human health. Increasing salt levels in freshwater systems favor salt-tolerant cyanobacteria, including some that can produce harmful toxins. Furthermore, rising salt levels have been linked to the increased release of toxins by cyanobacteria into the air and water. Consumption of or exposure to these toxins is known to cause liver damage and possible carcinogenic effects. Moreover, harmful blooms can adversely affect the tourism and food industries, causing additional economic hardship.
For our Urban and Ecosystem Ecology research topic, our group - Disease Detectives - wanted to investigate the effects of urbanization on coastal wetlands by analyzing the impacts of specific pollutants that enter coastal wetland waters on the biological communities found there. We also wanted to study the linkage between the above alterations in the survival and genetics of coastal wetland microorganisms and how they can potentially transmit vector-borne and water-carried human diseases. Finally, we wanted to evaluate the methods that can be used to measure and track the presence of these urban pollutants in coastal wetlands and how can we use this information to better preserve and improve the health and diversity of microbial communities
Research Question 1:
Does the runoff of road salts and de-icing agents into nearby coastal wetlands alter the survival of microorganisms such as phytoplankton and zooplankton?
Normally, the amount of Cl- concentrations in freshwater systems from natural sources are <20 mg/L, however this can increase to 1000s of mg/L due to use of road salts to clear snow and ice (Hintz & Relyea, 2019). It was shown that NaCl, the most commonly used de-icer, can decrease many bacterial densities within just 72 hours of exposure. It can also increase the diversity of denitrifying bacteria species in wetlands close to roads, which can alter the nutrient balance of the wetland (Hintz & Relyea, 2019). Moreover, zooplankton are microorganisms that are key players in freshwater systems as they graze and eat phytoplankton, and serve as prey for species of higher trophic levels. It was shown that zooplankton species like Daphnia decline after >200 mg/L, which cause the phytoplankton to become much more prevalent (Hintz & Reylea, 2019).
The study by Arnott et al. (2020) specifically conducted experiments on Daphnia (species of zooplankton) in a lake near a highway, and a lake >3 km away from roads where salt is applied in the winter, both of which reside in the Canadian Shield. It showed that Daphnia exposed to just 5 to 40 mg/L of chloride concentration through use of road salts had decreased reproduction and greater mortality, although this effect was not seen in the lake >3 km away from roads (Arnott et al., 2020).
Research Question 2:
How does the change in survivorship of microorganisms (i.e. phytoplankton and zooplankton) due to road salts and de-icing agents link to influence the transmission of vector-borne and water-carried human diseases?
The presence of road salts and de-icing agents entering wetland ecosystems not only affects the water, but also affects the surrounding communities who rely on this habitat to survive. An increase in salinity and chloride concentrations in coastal wetland ecosystems can affect microorganisms by altering their presence in the ecosystem. As mentioned in research question 1, there is a decrease in zooplankton along with an increase in phytoplankton. Due to less competition with zooplankton to consume phytoplankton, these wetlands become a harbour for mosquitoes, especially for Culex restuans, a mosquito vector for West Nile virus (Hintz & Reylea, 2019), contributing to the rise of vector-borne diseases in humans.
To provide an application of this research question, we also looked into the indigenous communities and their use of water from wetland ecosystems. We explored how these populations are interacting with urban pollutants and how specific contaminants affect their health. For instance, due to low levels of pathogen removal from wastewater, the presence of fecally derived microorganisms contributed to high incidences of gastrointestinal illness in an indigenous population in Nunavut, Canada (Daley et al., 2019).
Wastewater in Arctic communities is primarily treated through chlorination but leaves an abundance of pathogens in the water, which are released into wetland ecosystems. Indigenous populations interact with these environments and can become infected by the pathogens carried in the water. The vulnerability of drinking water to exposure of pathogens and other forms of contamination leads to the presence of waterborne disease in the North. In majority of communities within Arctic Canada, the most common waterborne illness is acute gastrointestinal illness (Harper et al. 2020). This arises due to the use of the wetland ecosystem for recreational and harvesting activities, with drinking water being identified as a greatest risk factor (Harper et al. 2020).
In many communities, surface water is either piped under- or above-ground to houses or is trucked to houses (Harper et al. 2020). As a result, heavy rainfall, rapid snowmelt, and climate-related events that increase water turbidity, can overwhelm water treatment infrastructure that is intended to protect public health (Harper et al. 2020). Furthermore, while most northern residents have access to treated tap water, the practice of drinking untreated water (e.g. from lakes, ponds, rivers, melted snow or ice) is quite common in these populations. This practice is incredibly unsafe due to intensified environmental change in water systems, increased water turbidity, and emergence of microbial and chemical contaminants (Harper et al. 2020). Drinking, bathing, washing, or eating food exposed to contaminated water can result in waterborne diseases and outbreaks that can be mild to life threatening.
Research Question 3:
What are some methods that can be used to measure and track the presence of road salts in coastal wetlands? How can we use this information to better preserve and improve the health and diversity of microbial communities?
How can benthic macroinvertebrates be used as a biological indicator to measure the level of ecological degradation of coastal wetlands due to road salts?
Macroinvertebrates are good indicators for pollutants in general as they have varying sensitivities to different types of pollutants. To answer question d) and use them to identify salt hotspots in wetlands, using them based on their biological traits rather than their taxa can provide more chemical-specific indication (Collin & Fahrig, 2020). Although there is less research available for this topic, there is an indication that macroinvertebrates can be used to evaluate the health of wetlands in terms of how polluted it is, what pollutants are present, and where these pollutants are located the most in wetlands (Collins & Fahrig, 2020). Preston et al. (2018) showed that brine (sodium chloride dominated water), specifically contaminated many coastal wetlands, negatively affecting the macroinvertebrate species inhabiting the area which are integral to the food web. In the study, the mean taxonomic richness, Shannon diversity, and Pielou evenness scores decreased for macroinvertebrates while percent domination increased in response to higher salinity (Preston et al., 2018). Again, although there is not enough literature that’s been published to identify the specific taxa that are consistently eliminated by brine due to confounding factors such as hydroperiod and natural salinity of the wetlands (Preston et al., 2018), this study indicates that there is a potential for macroinvertebrates to be used as indicators for the negative effects of salt contamination in wetlands.
What technology can be used to measure and monitor the levels of road salt contaminants and identify hotspot areas that contain these contaminants?
Monitoring road salt runoff in an urban setting can be quite difficult. People slipping on ice on private property leads to liability issues with property owners, who over-salt just to be safe (Smith, P., 2020, personal communication). Of course, monitoring road salt release on private property is pretty much impossible, so instead Ontario uses a provincial water quality monitoring network (Oswald, C., 2020, personal communication). This is a network of sites across the province where samples of water are collected and analyzed for different chemicals like chloride. Chloride sensors, which are small metal loggers with sensors that measure the electrical conductivity of water, can be used in urban areas that receive a lot of road salt. Programming these loggers to collect data every 15 minutes would be beneficial as it will allow us to see the chloride concentrations and how they fluctuate in the streams continuously. This can help demonstrate how chloride concentrations are changing in time and in response to changes in climate or management practices. These loggers can also be used to identify salt vulnerable areas if they are placed out in quite a few locations (Oswald, C., 2020, personal communication). How can we deal with pre-existing chloride pollution? Unfortunately, road salts are legacy pollutants, meaning the chloride we are measuring in wetlands could come from salt that was used many years ago, but depending on how it moved through the environment, only reached the wetlands now. Once road salts are on the landscape, we don’t have as much control of it anymore, especially because it comes from nonpoint sources (Oswald, C., 2020, personal communication). Further research is required to see if methods can be developed to clean the road salts pollution that have already reached the wetlands.
In terms of bioindicators, macroinvertebrates are suitable as they have varying sensitivities to different types of pollutants (Collin & Fahrig, 2020). A study by Preston and others (2018) showed that brine, which is sodium chloride dominated water, specifically contaminated many coastal wetlands, negatively affecting the inhabiting macroinvertebrate species which are integral to the food web. While the specific taxa that are constantly affected are not yet known, further research will help improve this indicator method.
Other methods to measure salinity include: soil sampling (involves collecting soil samples from wetlands and using calcium nitrate to extract chloride ions), vegetation sampling (which is when leaves and twigs of salt sensitive species, such as hemlock trees, are collected, dried and chloride concentrations are measured, this method can be useful for early detection of wetland salt contamination), and water sampling (which is when water is collected and chloride concentrations are measured) (Tharp & Allen, 2020).
Are there any alternative de-icing agents that can be used to reduce the amount of road salts being used?
Road salts like MgCl2 and CaCl2 are more effective than NaCl at colder temperatures, and so, reduce the amount of salt that is required per lane km (Schuler & Relyea, 2017). Provided that it is also more expensive, it is often mixed with other additives, such as beet juice. These additives also allow for the salt to stick to roads for longer periods of time, allowing less amounts of salt to be needed to replace the lost salts to continue clearing the roads of snow and ice.
The best thing to be doing is to use brines more, which are salty solutions. These are used for anti-icing, so before a storm would come, people would spray the brine like NaCl brine onto the road and it prevents the snow and ice from bonding to the pavement, reducing the amount of road salt needed to be out on afterwards. This is where there’s room to improve the use of brines, especially on private properties, which is a lot of the area that is salted. Using these are a good start. MgCl2 and CaCl2 are used for colder temperatures, but now there are 2 chlorides so we need to be careful about these (Oswald, C., 2020, personal communication).
Are there any foreseeable biological or economic problems surrounding the above solutions?
An article by Schuler and Relyea in 2017 looked at “GeoMelt” (MgCl2 with beet juice), and “Magic Salt” (MgCl2 premixed with a distillation by-product), and its effects on mosquitoes. Evidence was provided that since beet juice and distillation by-products contain phosphorus and carbohydrates, it acts as fertilizer that microbes feed on, which ultimately reduces the amount of dissolved oxygen (DO) in the water over time. This reduction in DO would affect many aquatic organisms, but would not impact mosquitoes since they breathe air (Schuler & Relyea, 2017). Moreover, the amount of phytoplankton was highest in the treatments with additives due to their available phosphorus that they can feed on. This decreased the emergence time for mosquitoes. It was also found that Culex restuans are capable of surviving in high concentrations of road salts, and also prefer to oviposit in more saline environments (Schuler & Relyea, 2017), all due to the reduction of zooplankton and increase in phytoplankton, as mentioned above.
These solutions can also change oxygen demand. A researcher at Canadian Center for Inland Waters (a federal research facility in Burlington) has been doing research on chloride impacts of freshwater mussels. She is looking at the effects of beet juice on organisms and is finding that the potassium levels are very high and can have a negative impact (Oswald, C., 2020, personal communication). People use cheese brine, and processed water from making beer, etc., but there needs to be research to see if there are other issues to using these products even if they are not chloride based (Oswald, C., 2020, personal communication).
What policies, both local (Canada) and global, are present to help conserve microbial communities and how much funding is available in these research areas to improve their health and diversity?
The Canadian Water Quality Guidelines mentions that the amount of chloride should not exceed 120 mg/L. There are chronic and acute thresholds that we want to stay below but there’s no regulation of road salting in Canada because it is needed to maintain road safety (environmental protection act for contaminants used to maintain road safety)
The federal government came up with a Code of Practice for environmental management of road salts - which only applied to public organizations that apply more than 500 tonnes annually. They make recommendations on developing salt management plans including information on application rates, and salt storage, and also ask organizations to identify salt vulnerable areas in their jurisdictions. But there is no enforcement of this. There is also a federal database, a voluntary database where municipalities are supposed to report salt usage. Some do and some don’t (Oswald, C., 2020, personal communication).
In terms of Cootes Paradise as a local case study:
-
Royal Botanical Gardens is the main organization in charge of the Cootes Paradise. However, the City of Hamilton (and sometimes provincial/federal government) is responsible for regulating the local wetlands, and so they are the main policymakers (Smith, P., 2020, personal communication).
-
Cootes Paradise is also a fish breeding area, and so the Ontario Ministry of Natural Resources and Forestry (Provincial) and Canada Ministry of Fisheries and Oceans (Federal) have potential roles to play as well (Smith, P., 2020, personal communication).
-
Funding for municipalities (who are the main players when it comes to road salt usage and regulation) is always short, and citizens don’t want to pay more taxes to fund for better technology etc. Funding will increase as municipalities begin to fully understand the issue and choose to act (Smith, P., 2020, personal communication).
References
Arnott, S.E., Celis-Salgado, M.P., Valleau, R.E., DeSellas, A.M., Paterson, A.M., Yan, N.D., Smol, J., & Rusak, J.A. (2020). Road salts impacts freshwater zooplankton at concentrations below current water quality guidelines. Environmental Science and Technology, 54(15), 9398-9407. doi: https://doi.org/10.1021/acs.est.0c02396
Collins, S. J., & Fahrig, L. (2020). Are macroinvertebrate traits reliable indicators of specific agrichemicals? Ecological Indicators, 111, 105965. doi:10.1016/j.ecolind.2019.105965
Hinsdale, J. (2018, December 11). How Road Salt Harms the Environment. State of the Planet, Columbia University. https://blogs.ei.columbia.edu/2018/12/11/road-salt-harms-environment/
Hintz W.D., & Relyea, R.A. (2019). A review of the species, community, and ecosystem impacts of road salt salinization in fresh waters. Freshwater Biology, 64(6), 1081-1097. doi: 10.1111/fwb.13268
Oswald, C. (2020, November 4). Road Salts and Microorganisms [Online interview]
Preston, T. M., Borgreen, M. J., & Ray, A. M. (2018). Effects of brine contamination from energy development on wetland macroinvertebrate community structure in the Prairie Pothole Region. Environmental Pollution, 239, 722-732. doi:10.1016/j.envpol.2018.04.088
Schuler, M.S., & Relyea, R.A. (2017). Road salt and organic additives affect mosquito growth and survival: an emerging problem in wetlands. Oikos, 127(6), 866-874. doi: https://doi.org/10.1111/oik.04837
Smith, P. (2020, November 7) Local Environmental Problems, Solutions, and Policy [Online interview]
Tharp, R., & Allen, D. (2020). Assessment of deicing salt storage and distribution as a salinization point source: The influence of permitting standards on water quality. Environmental Systems Research, 9(21), 1-15. https://doi.org/10.1186/s40068-020-00185-2